Simulation Examines Hidden Gas Turbine Mixing
Simulation Examines Hidden Gas Turbine Mixing
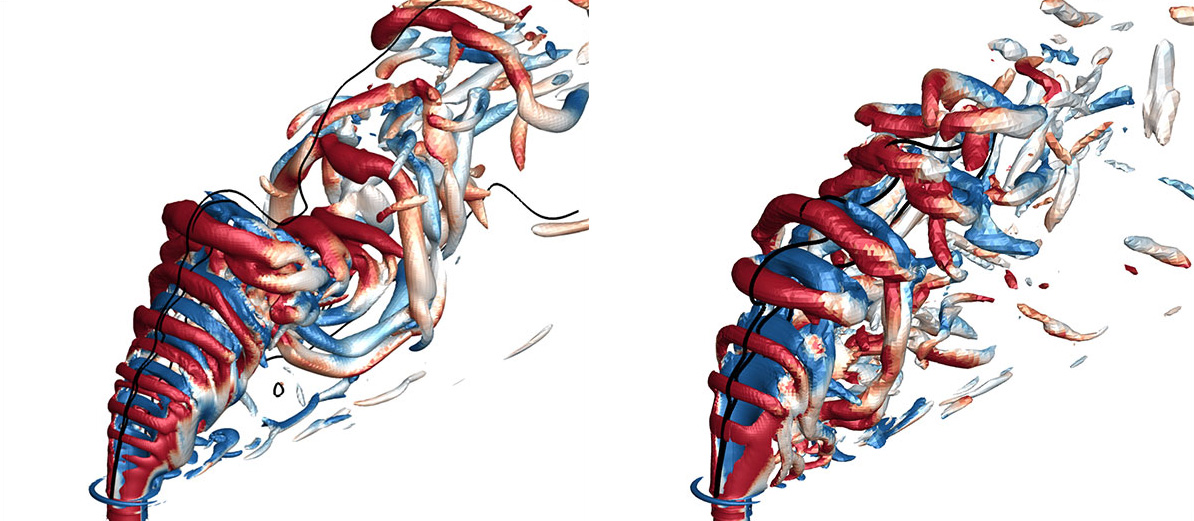
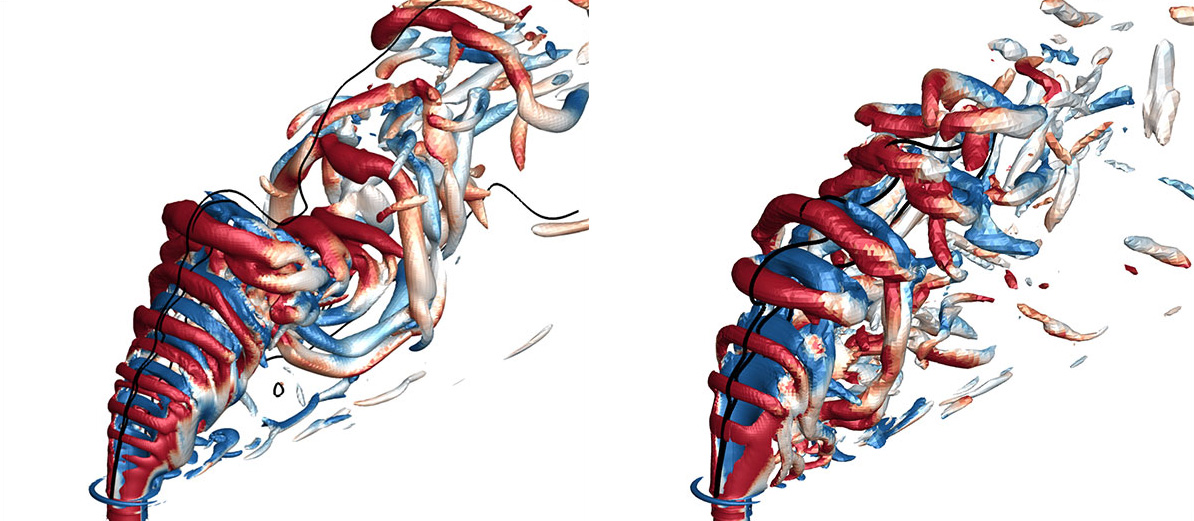
Researchers use two supercomputers to look at the way turbulence can change the way hot gases move through a turbine.
Gas turbines, such as jet engines on aircraft or the large machines that power many electric generating stations, look simple in the abstract. Fuel and compressed air ignite and rush through a set of blades that turn about an axis. But developing more advanced, more efficient turbines requires a far deeper understanding of the flow of combusted gases expanding through the blades.
Researchers at the Georgia Institute of Technology in Atlanta recently gained a better insight into the flow of gases by running a giant simulation on powerful computers at the San Diego Supercomputer Center in California and the Pittsburgh Supercomputing Center in Pennsylvania. The simulations of the jet shear layer, which is responsible for the formation of turbulent structures and vortices which control the mixing and the spreading of the combustion products, demonstrated that the layer can change quite suddenly inside the turbine under certain conditions. Those changes can lead to poor mixing and the production of pollution such as nitrogen oxides.
Become a Member
“Previous experimental work has shown that poor mixing in staged combustion systems leads directly to elevated NOx emissions. Essentially if the contents of the jet mix poorly with the crossflow gases prior to combustion, the heterogenous nature of the flow composition can result in local burning at unfavorable fuel-air ratios resulting in an increase in NOx,” said Vedanth Nair, a former research engineer at the Aerospace Combustion Lab at Georgia Tech who is now a lithography process engineer at Intel Corp. “Understanding how certain combustion configurations impact mixing would allow a more careful choice of operating conditions and design decisions.”
According to Nair, simulating an environment as complex as the inside of a gas turbine is a huge undertaking.
More Turbine Research: Novel Heat Treatment to Unlock High-Temperature, 3D-Printed Components
“Even simplifying the system to be studied, as done here, requires simulating fluid time scales on the order of tens of nanoseconds in order to capture the smaller scale fluid processes that govern mixing and influence combustion itself,” he said. On top of that, to be able to simulate the detail needed to see those processes requires more computing power than conventional computers can provide.
Both the Expanse machine at the San Diego Supercomputer Center and the Bridges-2 machine at the Pittsburgh Supercomputing Center are relatively new and have been designed for use in simulating everything from materials science to astrophysics.
One complication in understanding the processes inside a gas turbine is that during combustion, local regions of high-temperature gases and the energy given off by chemical reactions can significantly alter the organization of the flow through the turbine. Over the course of the simulation run, the Georgia Tech team found that the interaction between these elements and the flow of gases, known as the “flowfield,” can change more rapidly than was previously thought. What’s more, factors related to fluid viscosity and the location of the flame within gas turbine’s combustor all have great impact on the mixing of gases.
“This is increasingly critical when you consider next generation combustion systems, which can use highly reactive fuels such as hydrogen that behave considerably differently compared to natural gas,” Nair said.
The Georgia Tech team, which included Timothy Lieuwen, Vishal Acharya, Abin Krishnan, and Subodh Adhikari, published the results of their study in the American Institute of Aeronautics and Astronautics SciTech 2023 Forum Proceedings.
Read about the Gas Turbine Industry: Gaining Altitude
Part of the visualization produced by the supercomputers showed vortices—the swirling of gases in the flowfield as two separate streams of gases interact with one another—under different flow conditions. “Once these vortices are formed, they are further distorted by the interactions with the crossflow and further instability effects breaking them apart,” Nair said. “Visualizing the structure of these vortices provides valuable information on the impact of certain combustion effects on these instabilities and yields a qualitative idea of the mixing between the jet and the crossflow fluid.”
Nair said that one of the next steps will be to “simulate higher pressure flow conditions, as found in practical systems, which is challenging given the additional constraints on the numerical mesh and additional computational cost.”
Jeffrey Winters is editor in chief of Mechanical Engineering magazine.
Researchers at the Georgia Institute of Technology in Atlanta recently gained a better insight into the flow of gases by running a giant simulation on powerful computers at the San Diego Supercomputer Center in California and the Pittsburgh Supercomputing Center in Pennsylvania. The simulations of the jet shear layer, which is responsible for the formation of turbulent structures and vortices which control the mixing and the spreading of the combustion products, demonstrated that the layer can change quite suddenly inside the turbine under certain conditions. Those changes can lead to poor mixing and the production of pollution such as nitrogen oxides.
Become a Member
“Previous experimental work has shown that poor mixing in staged combustion systems leads directly to elevated NOx emissions. Essentially if the contents of the jet mix poorly with the crossflow gases prior to combustion, the heterogenous nature of the flow composition can result in local burning at unfavorable fuel-air ratios resulting in an increase in NOx,” said Vedanth Nair, a former research engineer at the Aerospace Combustion Lab at Georgia Tech who is now a lithography process engineer at Intel Corp. “Understanding how certain combustion configurations impact mixing would allow a more careful choice of operating conditions and design decisions.”
According to Nair, simulating an environment as complex as the inside of a gas turbine is a huge undertaking.
More Turbine Research: Novel Heat Treatment to Unlock High-Temperature, 3D-Printed Components
“Even simplifying the system to be studied, as done here, requires simulating fluid time scales on the order of tens of nanoseconds in order to capture the smaller scale fluid processes that govern mixing and influence combustion itself,” he said. On top of that, to be able to simulate the detail needed to see those processes requires more computing power than conventional computers can provide.
Supercomputing power
Both the Expanse machine at the San Diego Supercomputer Center and the Bridges-2 machine at the Pittsburgh Supercomputing Center are relatively new and have been designed for use in simulating everything from materials science to astrophysics.
One complication in understanding the processes inside a gas turbine is that during combustion, local regions of high-temperature gases and the energy given off by chemical reactions can significantly alter the organization of the flow through the turbine. Over the course of the simulation run, the Georgia Tech team found that the interaction between these elements and the flow of gases, known as the “flowfield,” can change more rapidly than was previously thought. What’s more, factors related to fluid viscosity and the location of the flame within gas turbine’s combustor all have great impact on the mixing of gases.
“This is increasingly critical when you consider next generation combustion systems, which can use highly reactive fuels such as hydrogen that behave considerably differently compared to natural gas,” Nair said.
The Georgia Tech team, which included Timothy Lieuwen, Vishal Acharya, Abin Krishnan, and Subodh Adhikari, published the results of their study in the American Institute of Aeronautics and Astronautics SciTech 2023 Forum Proceedings.
Read about the Gas Turbine Industry: Gaining Altitude
Part of the visualization produced by the supercomputers showed vortices—the swirling of gases in the flowfield as two separate streams of gases interact with one another—under different flow conditions. “Once these vortices are formed, they are further distorted by the interactions with the crossflow and further instability effects breaking them apart,” Nair said. “Visualizing the structure of these vortices provides valuable information on the impact of certain combustion effects on these instabilities and yields a qualitative idea of the mixing between the jet and the crossflow fluid.”
Nair said that one of the next steps will be to “simulate higher pressure flow conditions, as found in practical systems, which is challenging given the additional constraints on the numerical mesh and additional computational cost.”
Jeffrey Winters is editor in chief of Mechanical Engineering magazine.