Rethinking Classic Heat Transfer Theory
Rethinking Classic Heat Transfer Theory
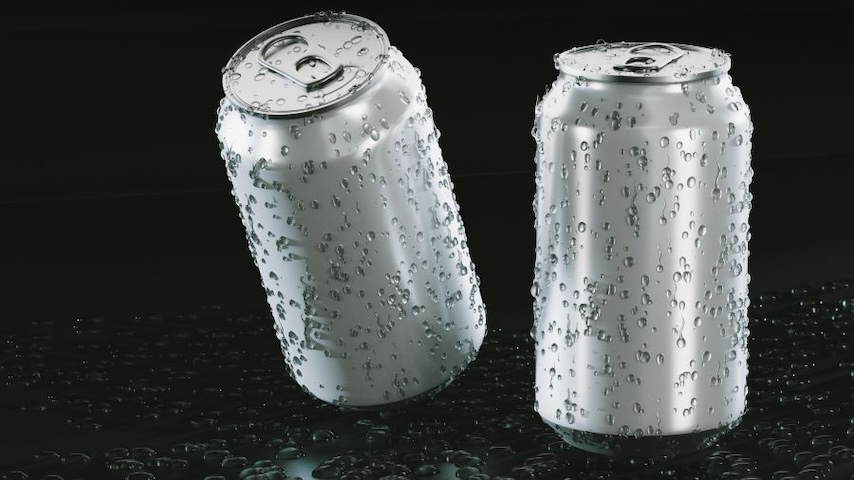
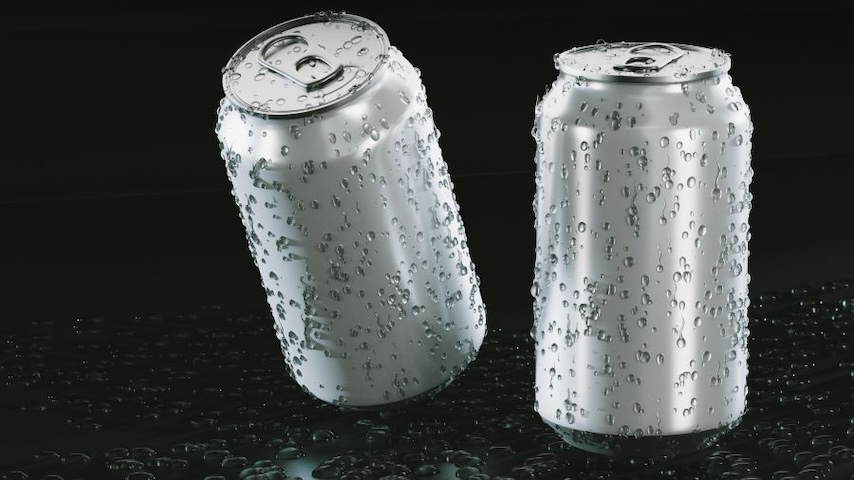
Remarkable heat transfer test results from a novel material leads University of Texas at Dallas researchers to challenge convention.
Any Physics 101 student can tell you that any mode of heat transfer is governed by temperature difference—and the larger that difference, the higher the heat transfer rate. Yet, in heat transfer phenomena like dropwise condensation, where vapor transforms into discrete droplets on a surface as opposed to a continuous film, other factors are also in play. Conventional models of this type of vapor-liquid phase change call for low contact angles and low contact angle hysteresis to get a high heat transfer coefficient. Now, researchers at the University of Texas at Dallas have found yet another variable that should be considered to optimize heat transfer: timescale.
Xianming (Simon) Dai, an associate professor of mechanical engineering in the Erik Jonsson School of Engineering Computer Science, said he and his colleagues were testing a quasi-liquid coating for a water harvesting application when they noticed something interesting. The material collected more condensates than expected.
“This coating is like a liquid for lubrication, but it’s not a liquid,” he explained. “Experimentally, it had a very high heat transfer coefficient. But when we looked back at the classic heat transfer model, which was developed more than half a century ago, it could not explain our results.”
The ultimate goal for Dai’s research team is to develop a unique surface that can collect and remove condensed droplets continuously in order to harvest water from the air without the aid of electricity. The mismatch in performance and the conventional physics model led the group to take a closer look at what might be happening. They used a time-resolved particle image velocimetry with a long-distance microscope to measure the activity of microscopic particles inside even the smallest harvested water droplets. The high resolution of the system allowed them to look at the flow velocity of small droplets as it rolled across the surface.
More for You: Better Airflow and Heat Transfer, Thanks to Dimples
“We saw that our unique quasi-liquid surface can promote droplet removal very fast,” said Dai. “The droplet is quickly rolling off the surface. The speed of disappearance, or removal of the droplets, is faster than the renucleation of new droplets.”
That disappearance speed was what hindered the classical model from explaining their observations. Because the formed droplets disappear “super fast,” the quasi-liquid coated surface looked as if there were no condensation droplets at all.
“How can we have heat transfer without condensation? Condensation from vapor to liquid must become a droplet,” he said. “Conventional surfaces show a slower disappearing rate than the growth rate of new droplets. You can see that droplet’s location and growth on the surface. The important factor here is timescale.”
Dai, along with research scientist Deepak Manga and Yaqing Jin, assistant professor of mechanical engineering, published a paper in the March 13, 2025, issue of Newton, proposing that the conventional model explaining vapor-liquid phase changes is limited and should include timescale in the future.
“Heat transfer theory does not account for the high speed at which this kind of surface can disappear condensates,” he said. “We need to redefine the model to guide our design for future summer energy systems so they can be more accurate and provide dynamic information about the droplet removal.”
Discover the Benefits of ASME Membership
When asked how this new model could help foster the design of more efficient heat transfer systems in the future, Dai said it could help create the kind of systems so more arid areas like West Texas can harvest water directly from the air.
“Around the globe, we have so many different energy systems. So many of them involve condensation, like heating, ventilation, and air conditioning [HVAC] systems,” he said. “Industrial heating processes also use condensation. This model could help improve energy efficiency around the globe as it informs new system designs.”
Dai hopes this addition to conventional heat transfer theory will help other engineers and scientists understand that new materials may require them to revisit classic theories and models of physics. No model is necessarily set in stone.
“These models may not be completely correct, or they may have limitations,” he said. “If you find results that may not make sense, it may be that you have uncovered a limitation. You can then do the experiments to see that and redefine the classic model so it can be leveraged for better design in the future. That is important for both the scientific and engineering communities.”
Kayt Sukel is a technology writer and author in Houston.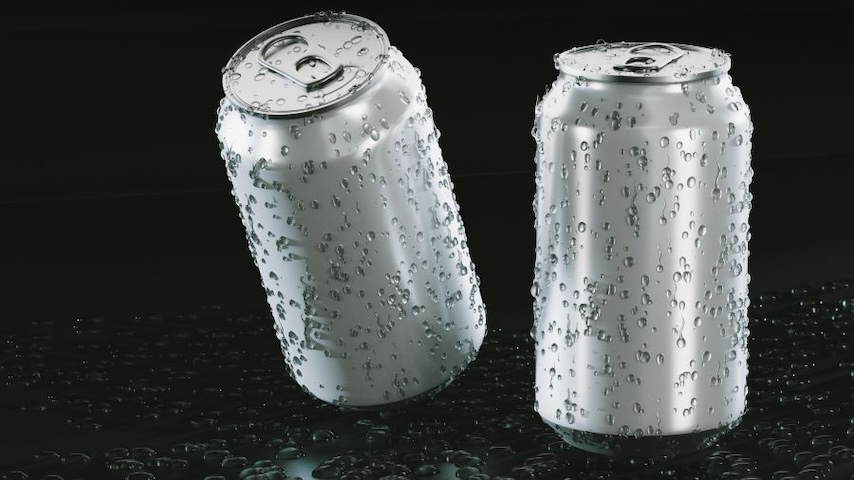
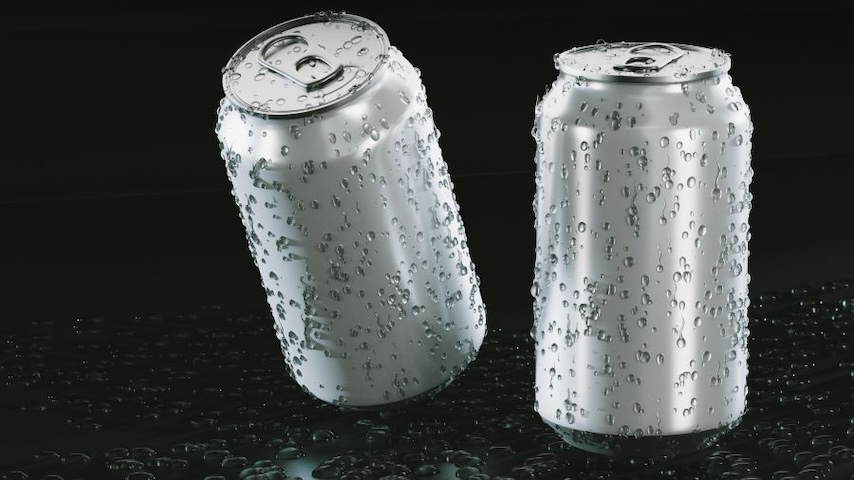